ADASOPS
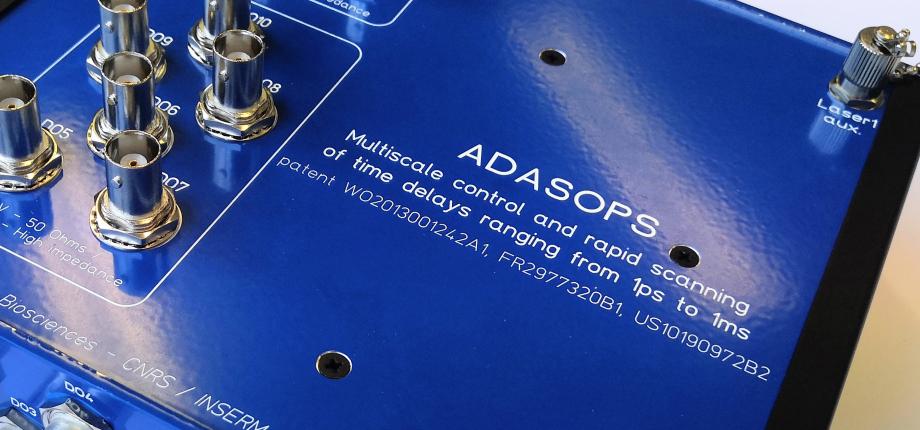
Arbitrary Detuning ASynchronous OPtical Sampling (ADASOPS)
Staff:
Laura Antonucci
Adeline Bonvalet
Manuel Joffre
Xavier Solinas
Ultrafast spectroscopy is based on the use of two pulses separated by a variable delay, the second pulse (probe) allowing to measure the modifications induced by the first one (pump) within the studied system, and to reconstruct point-by-point the dynamics of the system. Traditionally, the two pulses come from the same laser but travel different optical paths. The delay between the two pulses is obtained by mechanical displacement of the optics of one of the paths, as shown below. It is thus limited to the sub-nanosecond range: a delay beyond a few nanoseconds corresponds to mechanical displacements of more than one meter, making it difficult to maintain the pointing necessary for the experiment.
Fig. 1 : Conventional pump-probe experiment.
A more recent technique, called ASOPS (Asynchronous OPtical Sampling) requires two synchronized lasers, whose relative phase can be varied. We thus have two pulses separated by a variable delay, without mechanical translation, and with temporal excursion only limited by the period of the pump laser. Its major drawback is that it requires the frequency of one laser to be locked to the other, which implies modifications to the cavity of one laser and a sophisticated control system. It is also possible to use two amplified lasers, injected by two synchronised oscillators.
Fig. 2 : ADASOPS experiment.
The method we have developed also uses two lasers, but without any condition on their repetition rates, hence the acronym ADASOPS, for Arbitrary Detuning ASOPS. It allows to extend the use of ASOPS to any pair of femtosecond lasers, while keeping the advantages of the technique: no mechanical translation, high scanning speed, sub-picosecond temporal resolution, temporal excursion only limited by the pump laser period. This technique consists in measuring the repetition rates of the two lasers as well as the evolution of the relative offset between the two pulse trains. The delay between each pair of pulses can then be calculated a posteriori, and this with great precision thanks to the remarkable frequency stability of femtosecond oscillators. The determination of the relative evolution of the two pulse trains can be done either optically, by detecting with a photodiode the linear interference of two temporally overlapping pulses [1], or electronically, by detecting each pulse train and then performing a digital processing in an FPGA [2]. This latter approach, easier to implement, is the time-domain analog of super-resolution localization microscopy, where a large number of measurement leads to a great improvement in accuracy as compared to individual measurements. Applying an averaging algorithm implemented in a FPGA device we extract the evolution law with a resolution better than 200 fs [2].
A first variant, coined MHz ADASOPS, relies on two femtosecond oscillators. At LOB, we have developed a prototype with optical coincidence detection that has allowed us to reach a resolution of less than 400 fs over the entire 200 ns time domain corresponding to the period of our pump oscillator. This prototype was applied to the measurement of charge transfer within the photosynthetic reaction center of Rhodobacter Sphaeroides [3] and achieved a very good signal-to-noise ratio in only a few minutes. A crucial advantage of our method is to allow the use of a chirped pulse oscillator (CPO) as a pump laser, providing much more energy at a lower repetition rate.
A second variant, coined kHz ADASOPS, corresponds to the application of ADASOPS to amplified femtosecond lasers [4], as shown above in Fig. 2. The amplified pulses can also be selected to provide delays as close to the target values as possible, allowing both fast and logarithmic delay scans [5].
More recently we have developed a new prototype based on electronic measurements of the offsets between pump-oscillator pulses and probe-oscillator pulses.
The latest evolution of our ADASOPS prototype is shown in the figure below.
Fig. 3 : ADASOPS device, including an FPGA and a Time to Digital Converter.
ADASOPS is currently applied to a variety of multiscale pump-probe experiments, using either visible of mid-infrared probe pulses, in collaboration with Michel Sliwa and Marten Vos (see functional dynamics of biomolecules). An example of this collaboration is the use of visible pump / mid-IR probe spectroscopy for monitoring the dynamics of CO2 in the photoenzyme fatty acid photodecarboxylase, from picoseconds to hundreds of microseconds [6, 7].
The ADASOPS method is also being deployed with high-repetition rates Ytterbium lasers, within the ChirADASOPS ANR project lead by Pascale Changenet.
Publications
[1] L. Antonucci, X. Solinas, A. Bonvalet, M. Joffre, Asynchronous optical sampling with arbitrary detuning between laser repetition rates, Opt. Express 20, 17928-17937 (2012).
[2] L. Antonucci, X. Solinas, A. Bonvalet, M. Joffre, Electronic measurement of femtosecond time delays for arbitrary-detuning asynchronous optical sampling, Opt. Express 28, 18251-18260 (2020).
[3] L. Antonucci, A. Bonvalet, X. Solinas, M.R. Jones, M.H. Vos, M. Joffre, Arbitrary-detuning asynchronous optical sampling pump-probe spectroscopy of bacterial reaction centers, Opt. Lett. 38, 3322-3324 (2013).
[4] L. Antonucci, A. Bonvalet, X. Solinas, L. Daniault, M. Joffre, Arbitrary-detuning asynchronous optical sampling with amplified laser systems, Opt. Express 23, 27931-27940 (2015).
[5] X. Solinas, L. Antonucci, A. Bonvalet, M. Joffre, Multiscale control and rapid scanning of time delays ranging from picosecond to millisecond, Opt. Express 25, 17811-17819 (2017).
[6] D. Sorigué et al., Mechanism and dynamics of fatty acid photodecarboxylase, Science 372, No. 6538 (2021).
[7] A. Aleksandrov, A. Bonvalet, P. Müller, D. Sorigué, F. Beisson, L. Antonucci, X. Solinas, M. Joffre, Marten Vos, Catalytic mechanism of fatty acid photodecarboxylase: on the detection and stability of the initial carbonyloxy radical intermediate, Ang. Chem. Int. Ed. 63, e202401376 (2024).
Project funded by LabEx PALM & NanoSaclay in the Valorisation-2017 call for projects.